Research Topics
MEMS Reconfigurable Quantum Materials (MEGA-2D)
Collaborators: Prof. Yuan Cao, Prof. Amir Yacoby, Prof. Pablo Jarillo-Herrero, Prof. Shanhui Fan, Prof. Eric Mazur
Reconfigurable devices are gaining traction in the electronics and photonics communities, with multilayer materials like twistronics and cascaded metamaterials offering significant tunability. However, their full potential is often untapped due to challenges in modulating their multiple IDOFs. Micro(nano)electromechanical systems (M(N)EMS) technology stands out for its ability to finely tune optical devices, including sensors, modulators, and lasers. My postdoctoral work led to the development of a MEMS-integrated twistronics and twistoptics device, enabling precise adjustments of the interlayer gap and twist angle in photonic crystals or 2D materials.
-
In situ twisting and pressuring
-
Vdw force measurement
-
Nonlinear optical light source
-
Quantum light source
-
Strain effect
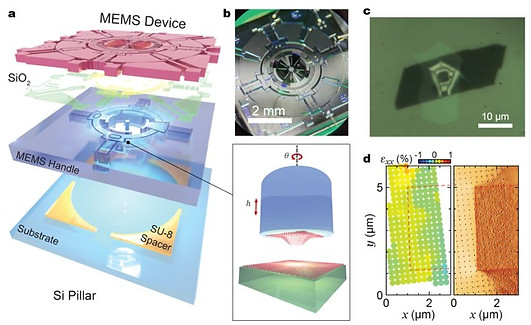
Figure 1: A key technique that I previous developed for this proposal is a MEMS-based on-chip device for tuning 2D materials, or MEGA2D for short. (a) A schematic of MEGA2D, allowing in situ tuning of distance, pressure, and twist angle between two 2D materials, one tented on a silicon pyramidal tip and the other flat on a substrate. (b) Photo of a complete MEGA2D device. (c) A zoom-in of two h-BN flakes controlled by MEGA2D. (d) Strain characterization of the h-BN on silicon pyramid, showing low residue strain <0.3%.
MEMS Reconfigurable Optical Metamaterials (MEGA-OM)
Collaborators: Prof. Eric Mazur, Prof. Shanhui Fan, Prof. Yuan Cao, Prof. Amir Yacoby, Prof. Evelyn Hu
The development of M(N)EMS optical metamaterials charts a path way towards versatile light manipulation and information processing especially using multi-IDoF bilayer devices, such as tunable chirality, spectrum and polarization, Purcell factor, structural light optical singularities, and tunable anisotropic plasmonic cavities. These scalable reconfigurable devices are robust across a wide temperature range, making them versatile for both research and practical applications in extreme conditions.
-
Arbitrary nonlinear optical tunability
-
Hyperspectral, hyperpolarimetric imaging
-
Chirality tuning
-
Tunable flat-band cavity
-
Single emitter selectivity
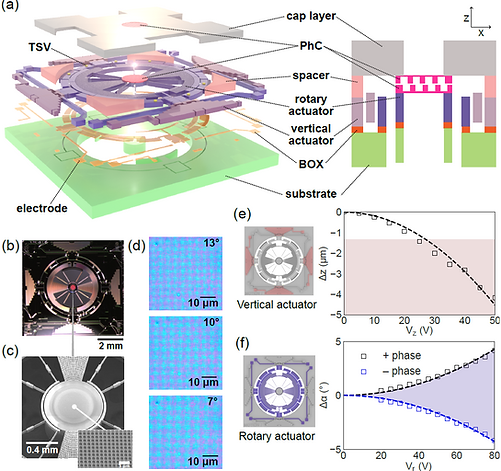
Figure 2: Microelectromechanically tunable twisted moiré photonic crystal sensor (a) Right: schematic of the primary components in the MEMS-TMPhC sensor. PhC = photonic crystal; TSV =through silicon vias; BOX = buffered oxide. Left: cross-sectional schematic (not to scale) of the MEMSTMPhCsensor integrating two photonic crystals (PhC) through monolithic fabrication. One of the two PhCs, can be moved vertically and rotationally by the MEMS actuators. (b) Microscope image of theMEMS-TMPhC sensor. (c) Scanning electron microscope image of the center of the MEMS-TMPhC sensor,showing one of the integrated PhCs (insert). (d) Microscopic image of the moiré pattern for different rotationangles. (e) Left: vertical actuator. Right: measured and fitted actuation curve for the vertical actuator. The shaded area indicates where a moiré pattern appears. (f) Left: rotary actuator. Right: measured andfitted actuation curve for the rotary actuator. The shaded area indicates the achievable angle range.
Twistoptics
Collaborators: Prof. Eric Mazur, Prof. Shanhui Fan, Prof. Evelyn Hu
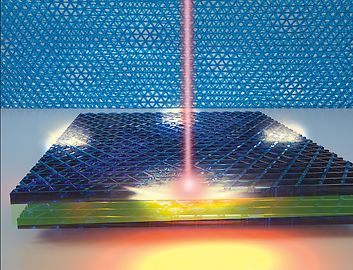
Figure 3: Schematic of twisted bilayer photonic crystals and the flat-band localization effect.
Moiré photonics leverages the twisting degree of freedom in photonic crystals to unveil unique optical properties such as directional scattering and advanced infrared sensing. My recent work has utilized this twist control in photonic crystal slabs, enabling extensive resonance tuning and pronounced circular dichroism in a compact form. This breakthrough paves the way for sensitive detection of both spectral and polarization changes, with the unexplored potential of topological twistoptics lying ahead.
My research investigates and generalizes the topological phases in quasicrystals to photonics, using computational methods and advanced fabrication tools, targeting the experimental realization of twist-dependent spatiotemporal band structures. I aim to explore the emergence of fragile topology in twisted bilayer photonic structures, inspired by similar discoveries in graphene. This research could unlock studies into "magnetic" photonic topology, usually exclusive to PT-symmetric crystals, and designs featuring higher-order topological charges. My findings suggest these structures can host adjustable spatiotemporal topological numbers, influenced by interlayer coupling. I'll investigate how twisting and shearing can create tunable spatiotemporal vortices, possibly leading to temporal singularities. This work promises new methods for manipulating light pulses for ultra-fast information technologies.
Zero-index metamaterials
Collaborators: Prof. Eric Mazur, Prof. Yang Li
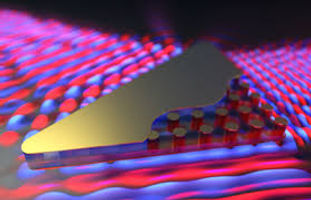
Materials with a zero refractive index support electromagnetic modes that exhibit stationary phase profiles. While such materials have been realized across the visible and near-infrared spectral ranges, radiative and dissipative optical losses have hindered their development. My research suggested ways to engineer the ultra-low-loss properties of the zero-index material, and the result led to the study of the many-body superradiance effect.
Figure 4: Schematic of a zero-index metamaterials.